REGISTRO DOI: 10.69849/revistaft/ch10202412141608
Andriela Dutra Norberto de Oliveira¹; Maria Alice Zarur Coelho²; Armando Lucas Cherem da Cunha²; Luis Gonzaga Santos Sobral¹; Isabella Teixeira Ribeiro de Oliveira²
ABSTRACT
The sulphide minerals bearing precious metals impose a real drawback for extracting them using the cyanidation process as they have to be oxidized in the first place, by the joint action of cyanide, the complex agent, and oxygen, the oxidizing agent, which consume cyanide before start dissolving the precious metals. One has also to consider that while dissolving the aforementioned sulphide minerals the ionic strength gradually increases turning the PGMs cyanidation process less efficient as the solubility of oxygen decreases as such. Therefore, this technical contribution aimed at using the bio-oxidation pre-treatment process to overcome such inconvenient making the precious metals extraction process more cost-effective.
Keywords: Sulphide minerals. Bio-oxidation. Cyanidation. Precious metals.
1. Introduction
Biohydrometallurgy is a high-value field of study within the metal extraction industry, emerging as a viable alternative to conventional extraction methods. This approach incorporates the use of microorganisms to obtain and recover metals from ores. Given the metabolic activities of these microorganisms, biohydrometallurgy has the potential to minimize environmental damage, operational costs, and harmful emissions associated with adverse techniques such as pyrometallurgy.
In this context, bio-oxidation, a biohydrometallurgical process, is characterized as a pre-treatment for concentrated and refractory ores. Microorganisms are utilized to oxidize mineral sulfides, thereby releasing gold encapsulated within the mineral structures into the solution (KAKSONEN et al., 2014).
According to Rodrigues (2016), numerous species of microorganisms with the ability to oxidize Fe²⁺ have been taxonomically identified. Examples include the bacteria Acidithiobacillus ferrooxidans, Leptospirillum ferrooxidans, and Acidithiobacillus thiooxidans (BRIERLEY, 2010; RODRIGUES, 2015; TAO and DONGWEI, 2014; WATLING, 2006), which are frequently employed in studies on biotechnological extraction processes.
In the bio-oxidation process for metals, microorganisms are responsible for generating the oxidizing agent in the reaction system (i.e., Fe³⁺ ions) through the oxidation of ferrous ions (i.e., Fe²⁺). These ions can be added to the system in either soluble form (FeSO₄·7H₂O) or insoluble form (FeS₂).
Based on the studies of Darvanjoobhi et al. (2022), bio-oxidation, one of the biomining methods alongside bioleaching, presents advantages when compared to other pyrometallurgical or hydrometallurgical pre-treatments, such as calcination and pressure leaching, respectively. The investment in reagents is reduced, and the process occurs under atmospheric pressure and mild temperature conditions, ranging between 30 and 50°C. These characteristics contribute to the recovery of desired metals within the required specifications while reducing environmental impact, ore costs, and waste stream management. However, the kinetics of this procedure are slow, requiring longer residence times compared to standard pre-treatment methods.
In the bio-oxidation process of iron-containing sulfides, such as pyrite, two simultaneous events occur: (i) the dissolution of sulfides results in the release of Fe and Ni, as well as the formation of SO₄²⁻, and (ii) the precipitation of secondary phases containing these elements. The formation of jarosite is frequently cited in the literature. In bio-oxidation systems, the precipitation of ferric compounds is strongly influenced by pH, temperature, and ferric ion concentration (HENAO, 2010). An increase in temperature or pH favors the formation of these precipitates. Therefore, these factors affecting the bio-oxidative process are regularly monitored.
In this study, the proposed objective was to investigate the bio-oxidation of gold ore as a pre-oxidative route for the mineral sulfides present, enabling the subsequent extraction of gold through chemical and electrolytic processes.
2. Experimental
The bio-oxidation assays were conducted in duplicate using Erlenmeyer flasks containing inorganic salts as nutrient sources: (i) (NH₄)₂SO₄ 80.0 mg·L⁻¹; MgSO₄·7H₂O 80.0 mg·L⁻¹; K₂HPO₄ 8.0 mg·L⁻¹, at pH 1.8; (ii) cultures of Leptospirillum ferrooxidans, Acidithiobacillus ferrooxidans, and Acidithiobacillus thiooxidans previously acclimatized; and (iii) ore. The flasks were incubated at a temperature of 30°C with orbital shaking at 150 rpm. Over the course of the 120-hour process, aliquots were collected every 24 hours for Fe and Ni analysis. Redox potential was monitored, and pH was recorded daily, with adjustments made using 5M H₂SO₄ as needed. The final pH was also recorded, and the volume of 5M H₂SO₄ used to adjust the pH in each Erlenmeyer flask was noted to evaluate acid consumption. At the end of the experiment, the supernatant was filtered, washed, and subjected to cyanidation tests.
For the gold extraction process, the material, after undergoing bio-oxidation as a pre-oxidative treatment, was placed in a glass reactor with a working volume of one liter (1000 mL) of aerated cyanide solution at varying free cyanide concentrations (i.e., this concentration depends on the mass of the gold ore being treated and its gold content, typically ranging from 3 to 10 g·L⁻¹, at pH 11). After cyanidation, the leachate was analyzed by ICP-OES to determine the extraction of gold and palladium. A direct cyanidation test was also conducted, where the ore was subjected to the same conditions without the bio-oxidative pre-treatment.
3. Results & discussion
By performing bio-oxidation as a pre-treatment for the ore subjected to cyanidation, the goal was to reduce the sulfide content to levels that would render the process more cost-effective. Figures 1 and 2 show that the extraction of nickel and iron increased over time during the bio-oxidation process.
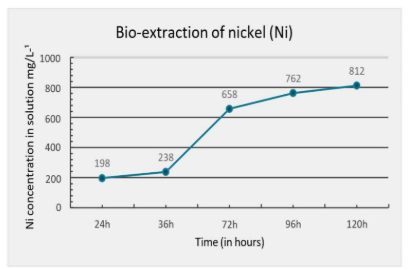
Figure 1 – Nickel Extraction Index Over the Duration of the Assay.
The results obtained (Figure 1) from the bio-oxidation process demonstrate a positive trend in nickel extraction over time. A consistent increase in the concentration of extracted nickel was observed, rising from 198 mg/L after 24 hours to 812 mg/L after 120 hours. This progressive increase suggests that the efficiency of nickel extraction improved over time.
The results for iron extraction (Figure 2) during the bio-oxidation process also show an increasing trend over time. The data indicate that the amount of extracted iron increased from 5.8 g/L after 24 hours to 7.4 g/L after 120 hours. However, a decline in the concentration of extracted iron was observed between 36 and 72 hours before it increased again. This fluctuation may be attributed to variations in process conditions, such as pH or temperature, which could have temporarily affected the efficiency of the bio-oxidation process during this period.
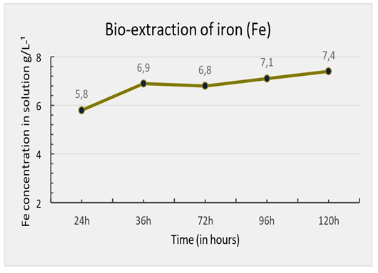
Figure 2 – Iron Extraction Index Over Time During the Assay
However, the microorganisms used in the process—Leptospirillum ferrooxidans, Acidithiobacillus ferrooxidans, and Acidithiobacillus thiooxidans—were not adapted to increasing ionic loads from the ore. Adapting the microorganisms to these conditions could potentially enhance nickel extraction efficiency, highlighting an area for improvement in future experiments.
Figure 3 presents the cyanidation results, showing a significant improvement in the extraction efficiency of gold (Au) and palladium (Pd) when bio-oxidation is applied as a pre-treatment prior to cyanidation. A progressive increase in gold recovery over time is observed, from 0.26 to 0.31, and finally to 0.36. This suggests that bio-oxidation facilitated more efficient leaching of gold. In contrast, direct cyanidation (Figure 4) shows a modest increase in gold recovery over time, from 0.12 to 0.13, and finally to 0.15. This indicates that direct cyanidation, without bio-oxidation pre-treatment, results in relatively low gold extraction efficiency.
Palladium recovery increased from 0.2 to 0.34 within three hours and reached 0.43 after six hours. While this represents an improvement, the efficiency is significantly lower compared to cyanidation following bio-oxidation.
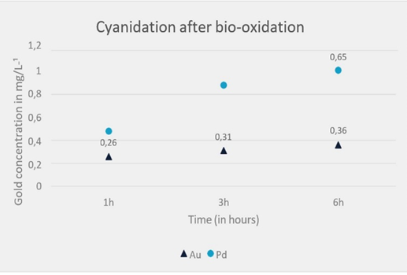
Figure 3 – Gold and Palladium Extraction Index Over Time Following Bio-Oxidation
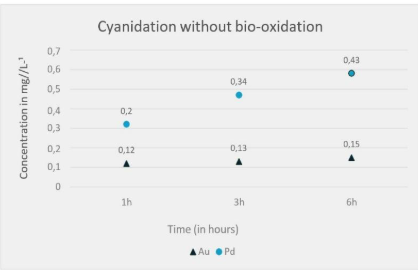
Figure 4 – Gold and Palladium Extraction Index Over Time Without Bio-Oxidation
The higher concentrations observed in the results following bio-oxidation can be attributed to the process’s ability to solubilize complex mineral sulfides that encapsulate precious metals. In the absence of bio-oxidation, these sulfides remain intact, limiting access to the precious metals and reducing the efficiency of cyanidation. Furthermore, direct cyanidation proves to be less cost-effective compared to cyanidation preceded by bio-oxidation..
However, incorporating a bio-oxidation stage significantly changes the scenario. The sulfides are oxidized, exposing the precious metals. This, in turn, makes cyanidation highly effective in extracting gold (Au) and palladium (Pd) from treated ores. Therefore, bio-oxidation as a pre-treatment stage significantly enhances the efficiency of precious metal extraction in industrial processes. This underscores the importance of bio-oxidation as a critical pre-treatment step for precious metal recovery.
4.Conclusions
Bio-oxidation has proven to be a crucial step for the efficient extraction of precious metals. By solubilizing complex mineral sulfides, bio-oxidation exposes precious metals, making them more accessible for subsequent extraction through cyanidation. The results indicate that the efficiency of gold and palladium extraction improved significantly when bio-oxidation was applied as a pre-treatment.
Furthermore, the absence of bio-oxidation resulted in relatively low extraction efficiency, as the sulfides were not initially solubilized, limiting access to the precious metals. This highlights the importance of bio-oxidation in enhancing cyanidation efficiency. Therefore, incorporating a bio-oxidation stage can be an effective strategy to improve the efficiency of precious metal extraction in industrial processes.
5. References
BRIERLEY, C. L. Biohydrometallurgical prospects. Hydrometallurgy, 2010; v. 104, p. 324–328.
CAPONERO, J. Reciclagem de Pneus. Doctoral Thesis, Escola Politécnica, Universidade de São Paulo, São Paulo, 2002.
DARVANJOOBHI, M. H. K.; MAGDOULI, S.; BRAR, S. K.; ABDOLLAHI, H.; ZOLFAGHARI, M. Bio-oxidation of gold from refractory sulfide ores: a journey ahead. Geomicrobiology Journal, v. 39, n. 3–5, p. 399–415, 2022. DOI: 10.1080/01490451.2021.1977431.
HENAO, D. M. O.; GODOY, M. A. M. Jarosite pseudomorph formation from arsenopyrite oxidation using Acidithiobacillus ferrooxidans. Hydrometallurgy, v. 104, n. 2, p. 162–168, 2010.
KAKSONEN, A. H.; MUDUNURU, B. M.; HACKL, R. The role of microorganisms in gold processing and recovery – a review. Hydrometallurgy, 2014. Accessed December 18, 2022.
RODRIGUES, M. L. M.; LOPES, K. C. S.; LEÔNCIO, H. C.; SILVA, L. A. M.; LEÃO, V. A. Bioleaching of fluoride-bearing secondary copper sulfides: Column experiments with Acidithiobacillus ferrooxidans. Chemical Engineering Journal, 2016.
RODRIGUES, M. L. M. Biolixiviação de cobre com Micro-Organismos Mesófilos e Termófilos Moderados: Sulfetos Secundários Contendo Flúor e Placas de Circuito Impresso. REDEMAT, UFOP, 2015. 116 p.
TAO, H.; DONGWEI, L. Presentation on Mechanisms and Applications of Chalcopyrite and Pyrite Bioleaching in Biohydrometallurgy – A Presentation. Biotechnology Reports, 2014.
WATLING, H. R. The bioleaching of sulfide minerals with emphasis on copper sulfides – A review. Hydrometallurgy, v. 84, p. 81–108, 2006.
1Metallurgical and Environmental Processes Coordination /CETEM – Centre for Mineral Technology, Brazil, Researchers
²School of Chemistry, Federal University of Rio de Janeiro, Brazil, Research Professors
Corresponding author: andriela.norberto7@gmail.com;